Research
Download SMSS Lab Brochure
The research at present is directed towards the following fields:
1. Dual Functional Metamaterials and Metastructures for Energy Harvesting and Vibration Control
The dynamics of periodic materials and structures have a profound historic background starting from Newton’s first effort to find sound propagation in the air to Rayleigh’s exploration of continuous periodic structures. This field of interest has received another surge from the early 21st century. Elastic mechanical metamaterials are the exemplars of periodic structures that exhibit interesting frequency-dependent properties like negative Young’s modulus and negative mass in a specific frequency band due to additional feature of local resonance. It implies, the spatial periodicity of mechanical unit cells in engineered metamaterials exhibits properties beyond one can expect from conventional naturally occurring materials. Locally resonant units in the designed metamaterial facilitate bandgap formation virtually at any frequency for wavelengths much higher than the lattice length of a unit cell. Whereas at higher frequencies for wavelengths equal to the lattice size of the medium, the Bragg scattering phenomenon occurs, which also helps in the bandgap formation. Due to out of phase motion of multiple resonating units with lattice, there is a change in the dynamic behavior (stiffness or mass) of the material as physical properties become frequency-dependent.
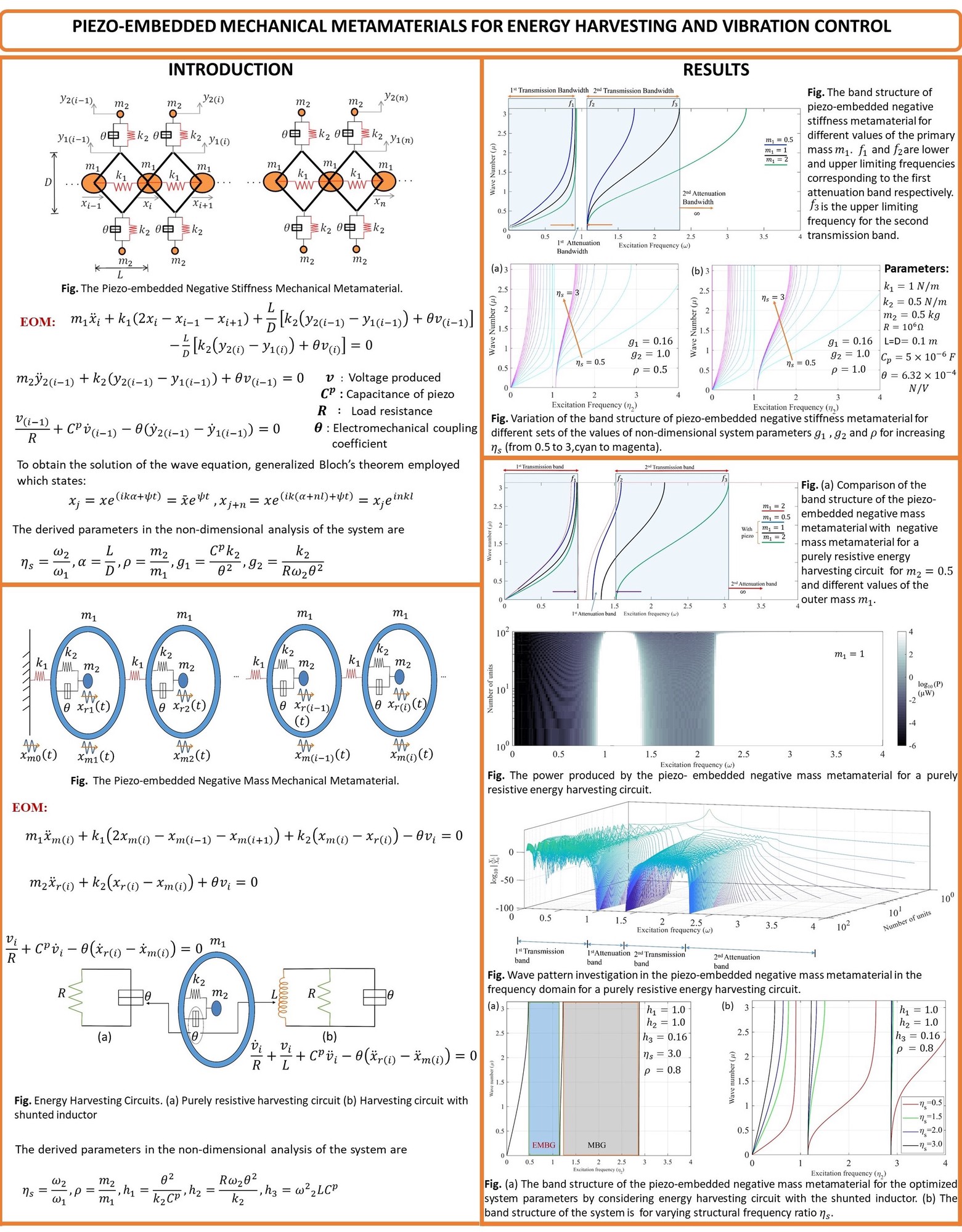
Consequently, these extreme frequency-dependent physical properties modulate wave propagation through designed metamaterials and generate attenuation bands. Bloch’s theorem is used to solve periodic structure problems in various fields. This theorem gives the relationship between frequency and wave number, and subsequently, the band structure of piezo-embedded mechanical metamaterials can be obtained. Successively, harvested power and transmissibility can also be computed for a chain of a finite number of metamaterial units by using the backward substitution method. The addition of the piezoelectric material at the resonating unit increases the damping and complexity of the solution. The insertion of the piezoelectric material in the resonating unit provides better tunability of the system. By identifying critical parameters through an extensive non-dimensional study of this system, the designed metamaterial band structure can be tailored. It enhances the performance in terms of vibration attenuation and harvested energy. This research can be considered as the first step towards designing the active elastic mechanical metamaterials.
Reference:
2. Exploring the dynamics of hourglass shaped lattice metastructures
Continuous demand for the improvement of mechanical performance of engineering structures pushes the need for metastructures to fulfil multiple functions. Extensive work on lattice-based metastructure has shown their ability to manipulate wave propagation and producing bandgaps at specific frequency ranges. Enhanced customizability makes them ideal candidates for multifunctional applications. This paper explores a wide range of nonlinear mechanical behavior that can be generated out of the same lattice material by changing the building block into dome shaped structures which improves the functionality of material significantly. We propose a novel hourglass shaped lattice metastructure that takes advantage of the combination of two oppositely oriented coaxial domes, providing an opportunity for higher customizability and the ability to tailor its dynamic response. Six new classes of hourglass shaped lattice metastructures have been developed through combinations of solid shells, regular honeycomb lattices and auxetic lattices. Numerical simulation, analytical modelling, additive layer manufacturing (3D printing) and experimental testing are implemented to justify the evaluation of their mechanics and reveal the underlying physics responsible for their unusual nonlinear behaviour. We further obtained the lattice dependent frequency response and damping offered by the various classes of hourglass metastructures. This study paves the way for incorporating hourglass based oscillators to be used as building block of future mechanical metamaterials, leading to a new class of tunable metamaterial over a wide range of operating frequencies. The proposed class of metastructure will be useful in applications where lightweight and tunable properties with broadband vibration suppression and wave attenuation abilities are necessary.
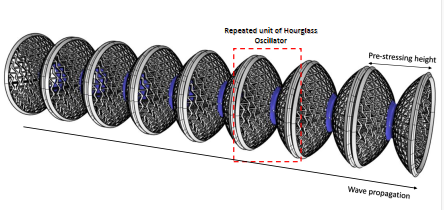
Reference:
3. Metamaterial inspired tensairity beam for frequency band attenuation
Tensairity refers to a class of lightweight structure which has a wide range of interesting applications such as temporary bridges, inflatable kites, of unmanned aerial vehicle wings and mainsail in sailing boats. A Tensairity structure has three main components, namely tension element, compression element and air beam. The primary purpose of the air beam is to stabilise the compression element under loading. The combination of these elements results in a structure which has lightweight compared to conventional structures for the same strength and vice versa. In this study, we explore a new concept of meta tensairity beam.
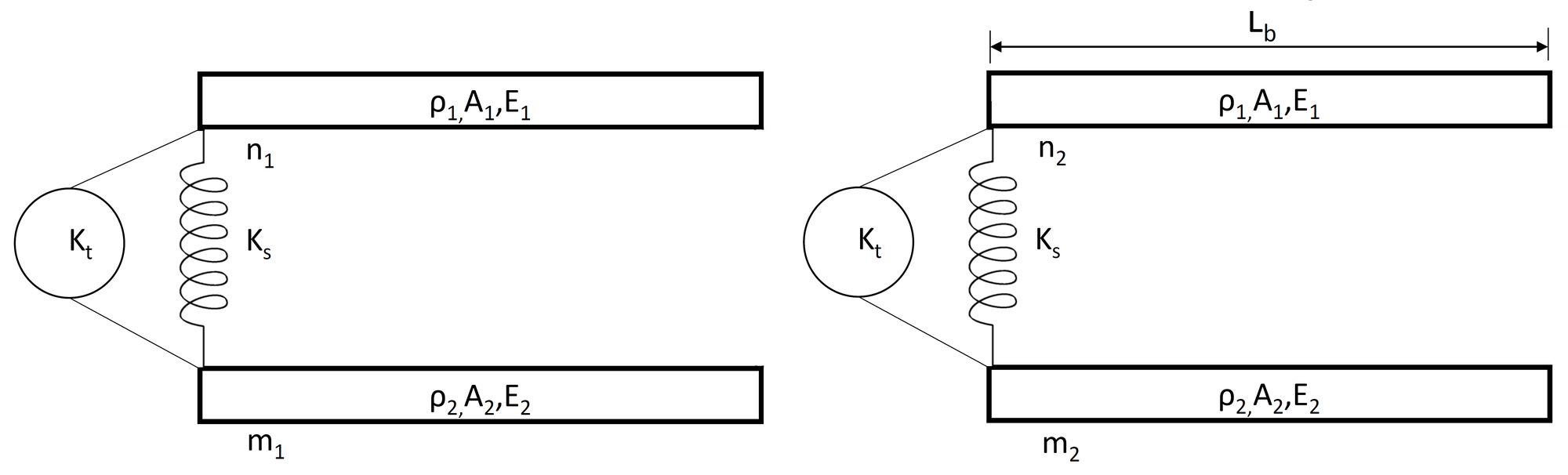
Air is modelled as spring, and an additional torsional spring has been used between the two beams and this structure has been repeated periodically. Both tension and compression elements have been modelled as Euler Bernoulli beam. The unwanted vibration which occurs in the tensairity structure can be attenuated by varying the stiffness of torsional spring. Band structure of meta tensairity beam has been obtained by using Bloch theorem and transfer matrix method. The phenomenon of frequency band attenuation has been incorporated in the Tensairity structure, and it gives rise to a new set of design potentials for lightweight structures. Real-time health monitoring of tensairity structures can also be done by harvesting energy from meta tensairity, which makes it a self-sustaining system.
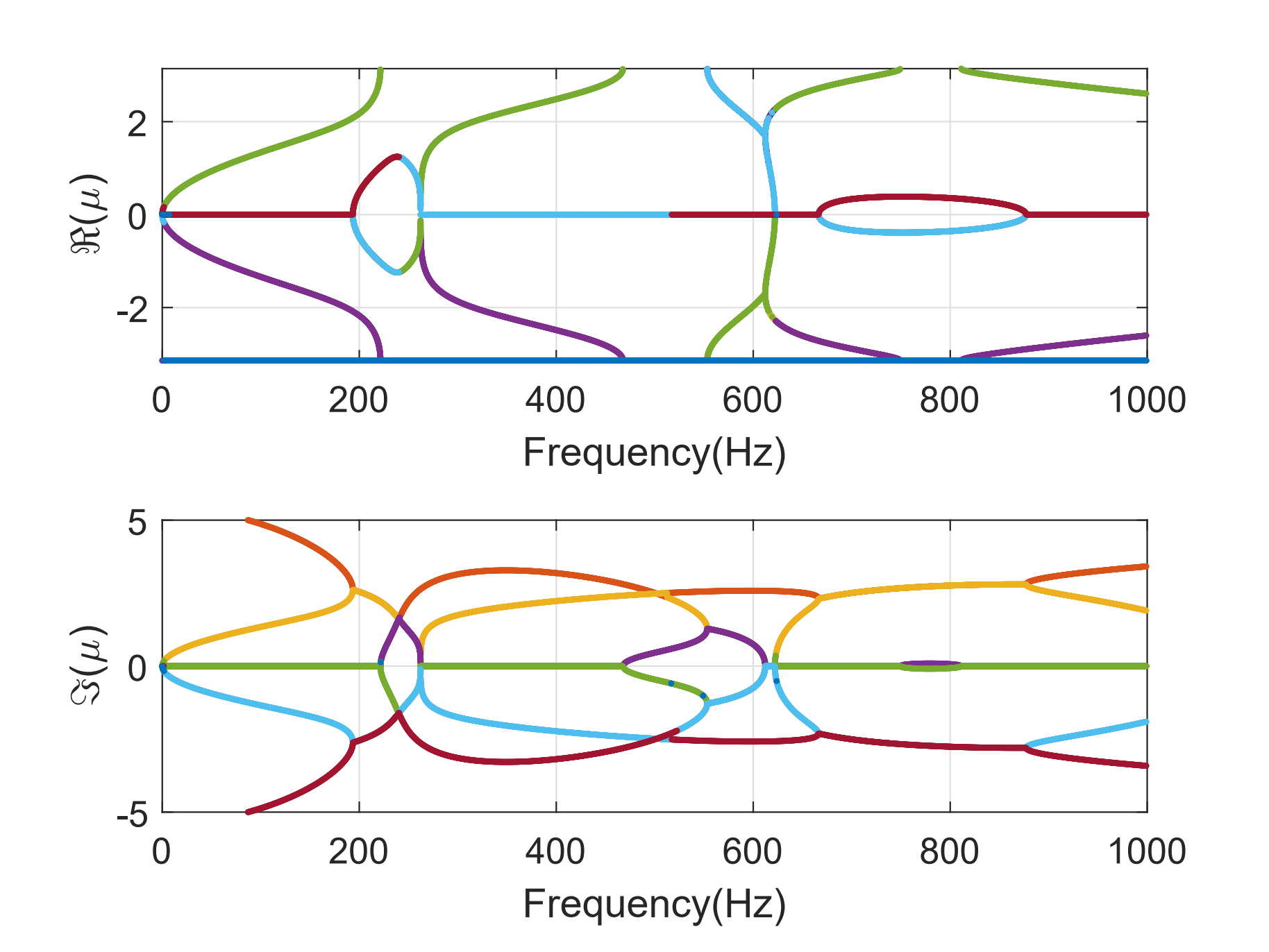
Reference:
4. Voltage modulated elastic moduli in lattice metamaterials
Two-dimensional lattices are ideal candidate for developing artificially engineered materials and structures across different length-scales, leading to unprecedented multi-functional mechanical properties which can not be achieved in naturally occurring materials and systems. Characterization of effective elastic properties of these lattices is essential for their adoption as structural elements of various devices and systems. An enormous amount of research has been conducted on different geometry of lattices to identify and characterize various parameters which affect the elastic properties. However, till date we can not control the elastic properties actively for a lattice microstructure, meaning that the elastic properties of such lattices are not truly programmable. All the parameters that control the effective elastic properties are passive in nature. After manufacturing the lattice structure with a certain set of geometric or material-based parameters, there is no room to modulate the properties further.
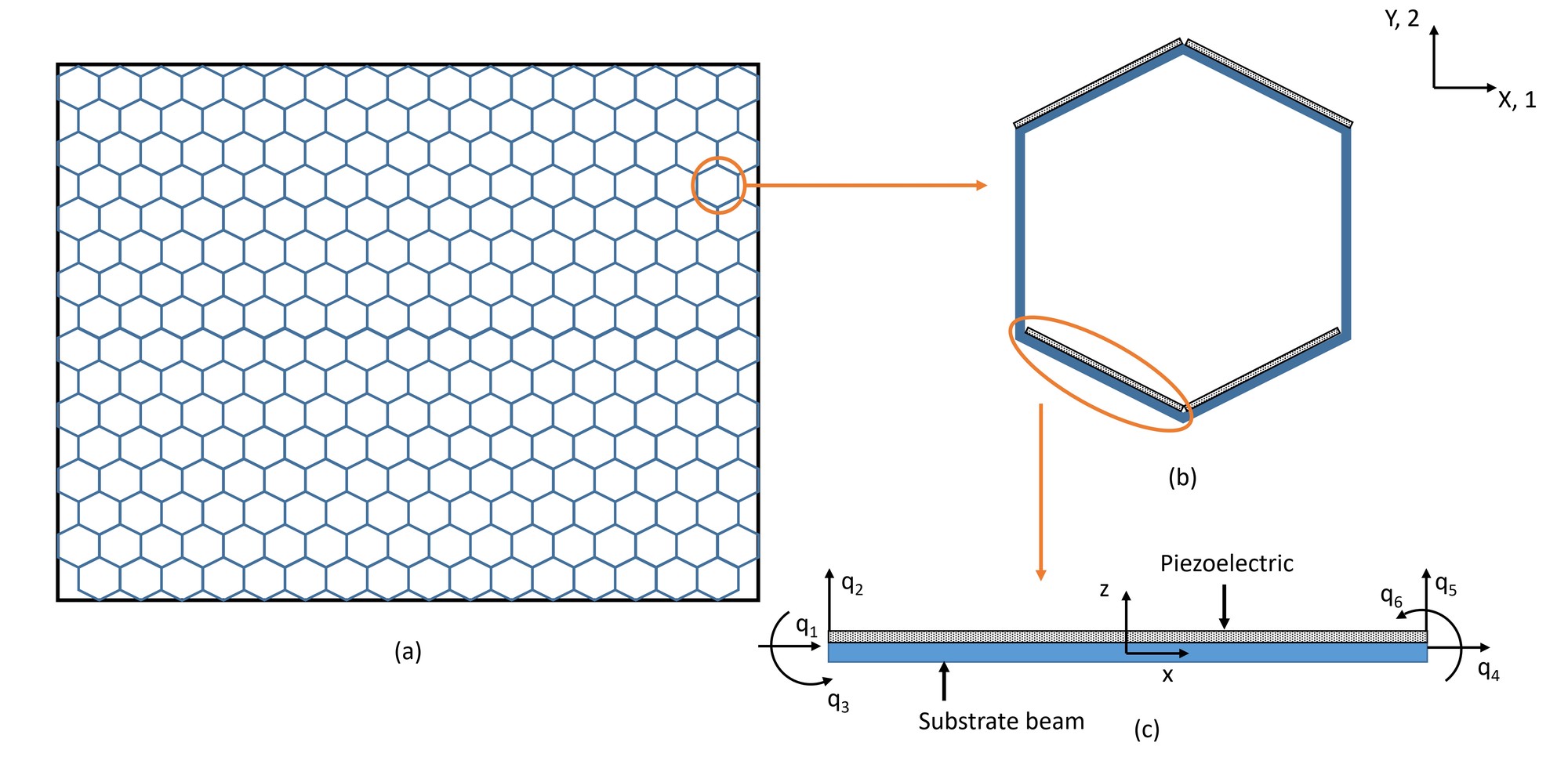
In this article, we propose a hybrid lattice micro-structure by integrating piezo-electric materials with the members of the lattice for active voltage-dependent modulation of elastic properties. A bottom-up multi-physics based analytical framework leading to closed-form formulae is derived for hexagonal lattices to demonstrate the concept of active lattices. It is noticed that the Young’s moduli are voltage-dependent, while the shear modulus and the Poisson’s ratios are not functions of the applied voltage. Thus, the compound mechanics of deformation induced by external mechanical stresses and electric field lead to an active control over the Young’s moduli as a function of voltage. Interestingly, it turns out that a programmable state-transition of the Young’s moduli from positive to negative values with a wide range can be achieved in such hybrid lattices. The physics-based analytical framework for active modulation of voltage-dependent elastic properties on the basis of operational demands provide the necessary physical insights and confidence for potential practical exploitation of the proposed concept in various futuristic multi-functional structural systems and devices across different length-scales.
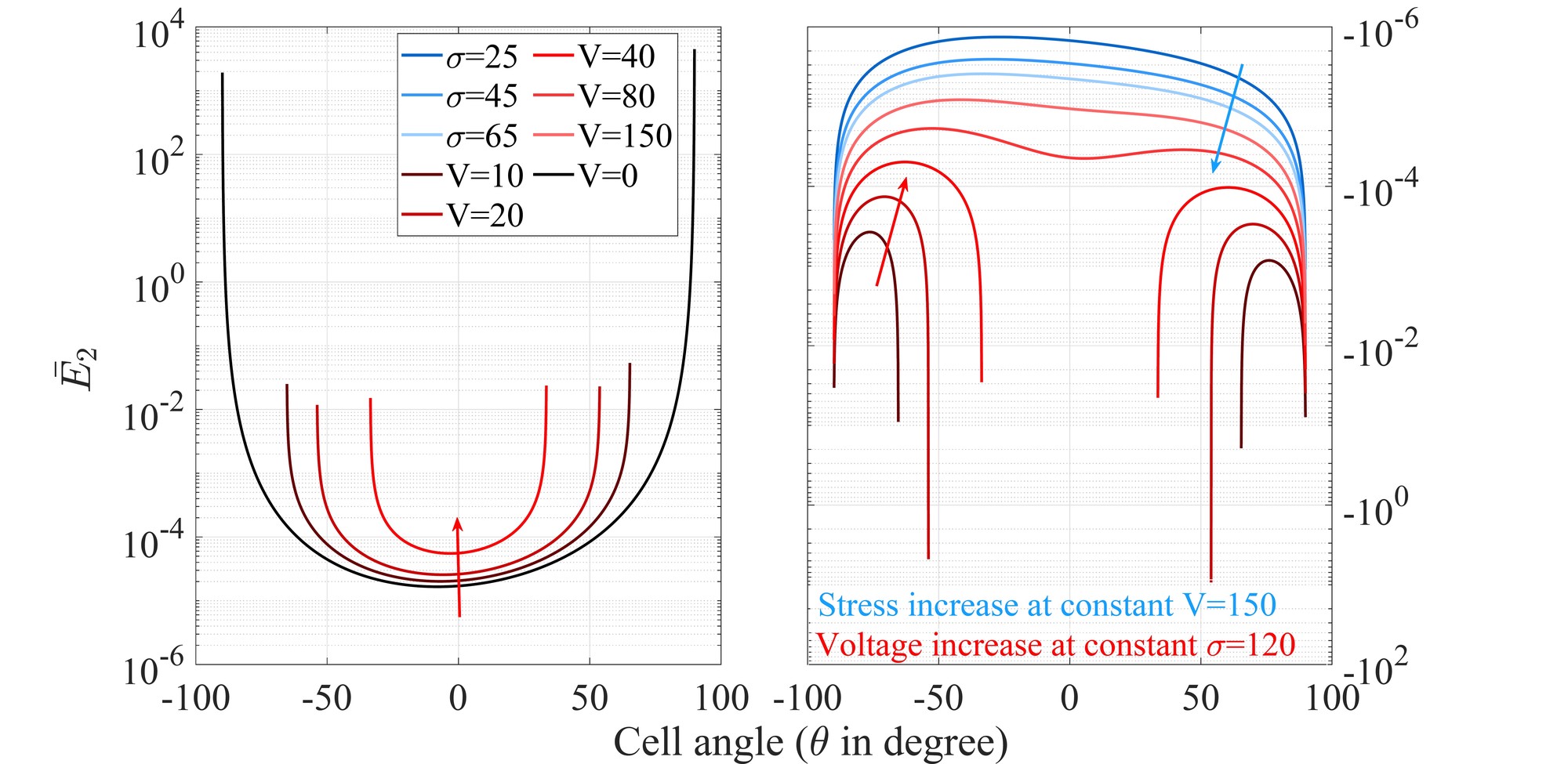
Reference:
5. Energy Harvesting System
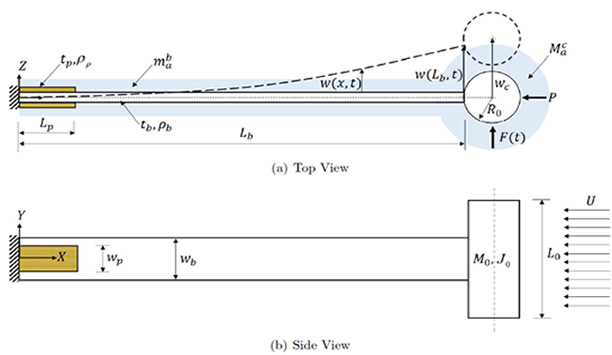
Bluff bodies like circular cylinders when placed in moving fluid shed vortices. These vortices apply a harmonic force on the cylinder. If the cylinder is allowed to move freely, it vibrates and itself exerts a force on the fluid giving rise to a fluid-solid interaction. Large amplitude vibrations occur when the frequency of vortex shedding becomes equal to the natural frequency of the structure. Flow of water in rivers can act as an ambient source of vibration on spring mounted cylindrical bluff body by subjecting it to vortex-induced vibrations. A cantilever beam having a cylindrical tip mass and a piezoelectric element as transducer can form an energy harvesting system for micro-power generation. The cylindrical tip mass acts a bluff body and sheds vortices thereby vibrating the cantilever beam. At the fixed end of the cantilever beam, a piezoelectric composite patch is attached which converts the mechanical energy coming from bending to electrical energy. Such a system can be one of the ways to power the sensors for an Autonomous Aquatic Observatory that monitors the health of aquatic bodies like river. VIV can be modelled by coupling two oscillators, a harmonic oscillator for structural vibration and a Van der Pol oscillator for modelling the dynamics of vortex-shedding. The couplings can be solved to obtain the response of the system. The VIV based EH system also forms a complex multi-parameter problem in which optimisation techniques may also be implemented to enhance the power generation capability.
6. Shape Memory Alloy-Based Sensor for Two Phase Flow Detection
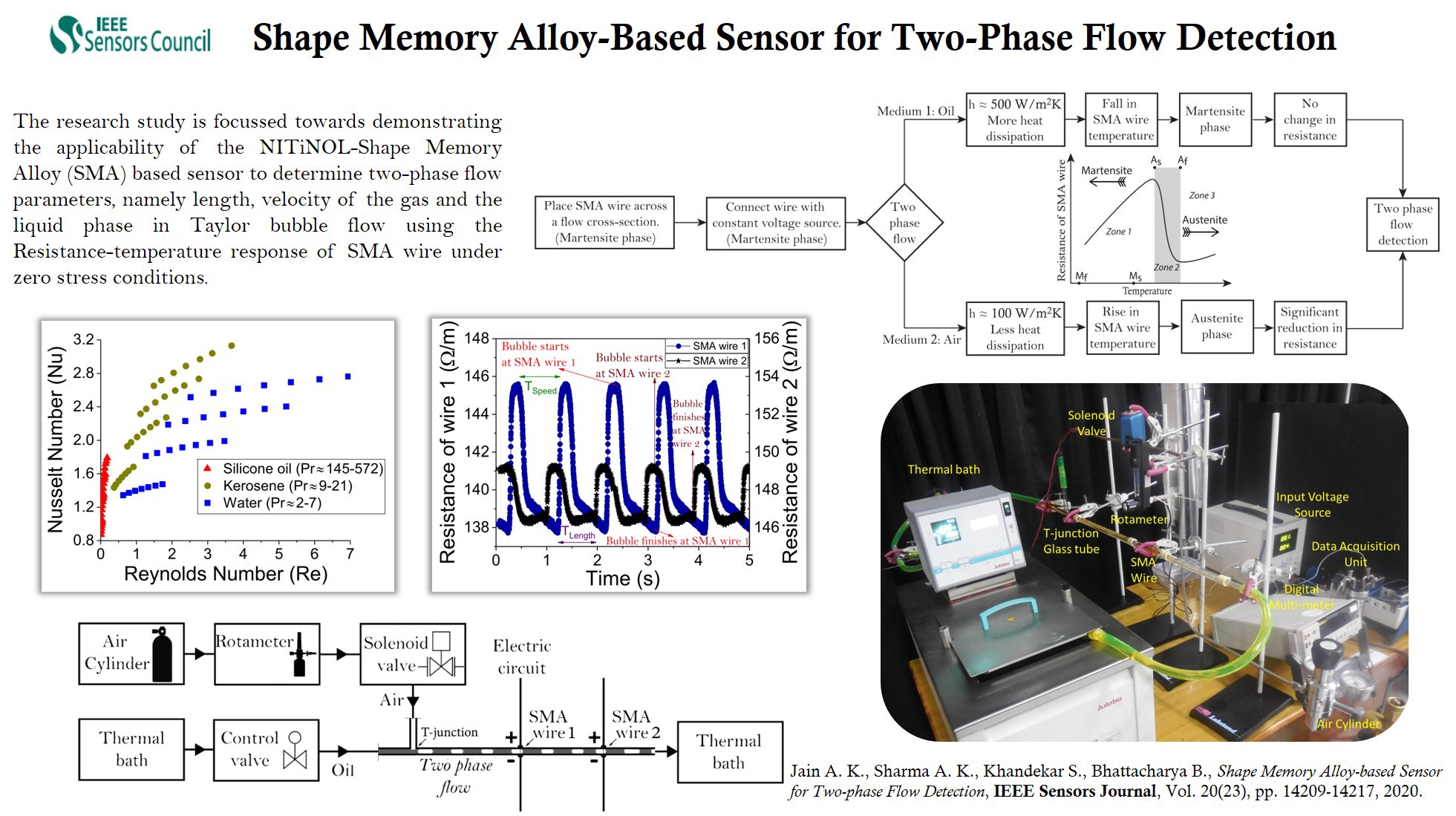
7. Energy Harvesting and Health Monitoring of Laminated Composites using Embedded Smart Sensors
Delamination and defects in laminated composites does not affect the free vibration properties significantly. However, embedded magnetostrictive layers are found to be affected by such change due to the variation of in-plane deformation. A suitable instrument from outside the structure could pick up this change as a voltage signal. The magnetostrictive layers would undergo a change in deformation generating small electromagnetic field, which could be sensed by Gauss meter etc. The current research is directed to the numerical study of such phenomenon using high precision smart finite elements. One of the problems of active signal processing is the necessity of power supply from outside the structure. In the current research, motes (MEMS prototypes) powered by structural vibration is used for self actuation and sensing.
8. Study of Vibration Damping in Particulate Composites
Particulate composites with smart piezoelectric particles deposited in conductive resin, is considered for vibration damping application. This is a passive damping technique, which can transform the mechanical energy in vibration into electrical energy and thereby enhance energy dissipation. Different Perovskite ceramic particles are chosen for preparing the particulate composite. Currently, the effects of shape, size and volume fraction of the particles on vibration damping are being studied. The experimental as well as numerical studies are carried out initially only on different particulate composite beams. The effect of solid-solid and solid ?viscoelastic interaction in unit cell system has been numerically modeled and the possible material loss-factors with respect to different excitation frequencies have been evaluated. The method will be farther extended to composite structures. The author is the principal investigator of a MHRD project on Smart Prosthetic Limb in which the research output will be directly applied.
9. Development of Flexible Manipulator for Micro-Flying Robots
Following a bio-simulated study, wings of micro-flying robots are being designed which can generate significant lift using power of milli-watt level. Thin composite plates of different shapes and sizes are being developed and patches of smart layer are bonded to the thin wings. The low frequency dynamic characteristics of the wings are then studied in a smart plate test bench. The experiments will be now carried out inside a wind-tunnel where the wings will be subjected to different flow patterns and the PIV (particle image velocimetry) technique will be used to compare the aerodynamic performances corresponding to different morphologies of the wing.